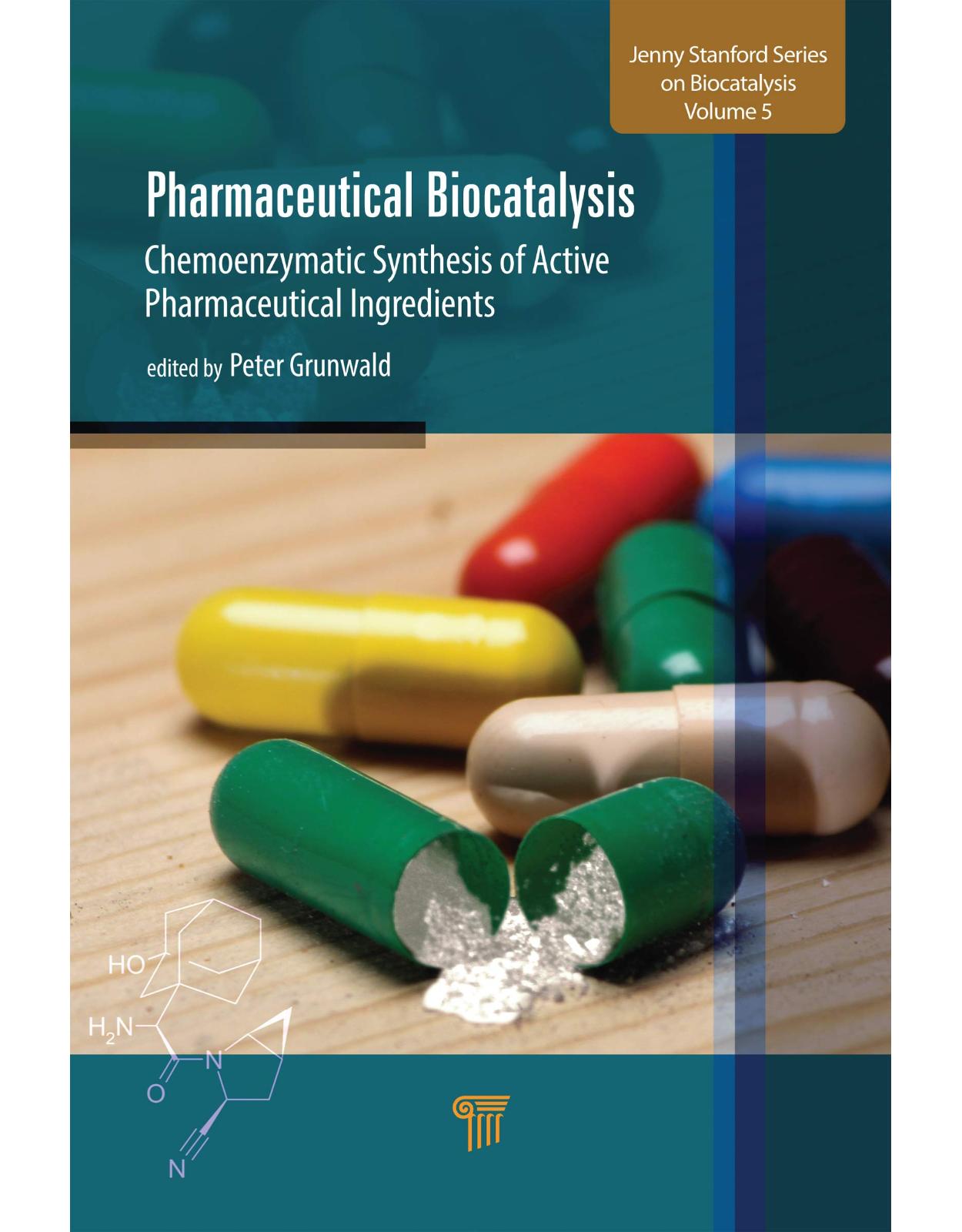
Pharmaceutical Biocatalysis: Chemoenzymatic Synthesis of Active Pharmaceutical Ingredients
Livrare gratis la comenzi peste 500 RON. Pentru celelalte comenzi livrarea este 20 RON.
Disponibilitate: La comanda in aproximativ 4 saptamani
Autor: Peter Grunwald
Editura: CRC Press
Limba: Engleza
Nr. pagini: 862
Coperta: Hardcover
Dimensiuni: 15.49 x 4.06 x 23.11 cm
An aparitie: 8 Jan. 2020
Description:
This volume provides an insight into the future strategies for commercial biocatalysis with a focus on sustainable technologies, together with chemoenzymatic and biotechnological approaches to synthesize various types of approved and new active pharmaceutical ingredients (APIs) via proven and latest synthetic routes using single-step biocatalytic or enzyme cascade reactions. Many of these drugs act as enzyme inhibitors, as discussed in a chapter with a variety of examples. The targeted enzymes are involved in diseases such as different cancers, metastatic and infectious diseases, osteoporosis, and cardiovascular disorders. The biocatalysts employed for API synthesis include hydrolytic enzymes, alcohol dehydrogenases, laccases, imine reductases, reductive aminases, peroxygenases, cytochrome P450 enzymes, polyketide synthases, transaminases, and halogenases. Many of them have been improved with respect to their properties by engineering methods. The book discusses the syntheses of drugs, including alkaloids and antibiotics, non-ribosomal peptides, antimalarial and antidiabetic drugs, prenylated xanthones, antioxidants, and many important (chiral) intermediates required for the synthesis of pharmaceuticals.
Table of Contents:
1. Future Strategies for Commercial Biocatalysis
1.1 Introduction
1.2 Cascades
1.2.1 Cell-Free Enzyme Cascades
1.2.2 Chemoenzymatic Cascades
1.3 Micro- and Nanoscale Process Design Considerations
1.3.1 Nanoscale Compartmentalisation
1.3.2 Microfluidic Reactors
1.4 Conclusion
2. Synthetic Approaches to Inhibitors of Isoprenoid Biosynthesis
2.1 Introduction
2.2 Bisphosphonates
2.2.1 Direct Method: Reaction of Carboxylic Derivatives with Phosphorous Reagents
2.2.2 Indirect Method: Reaction of Acylphosphonates with Dialkyl Phosphites
2.2.3 Michael Addition to Tetraethyl Vinylidenebisphosphonate
2.2.4 Alkylation of Tetralkylbisphosphonate
2.2.5 Other Methods
2.3 Non-Bisphosphonate Derivative
2.4 Concluding Remarks
3. Using a Recombinant Metagenomic Lipase for Enantiomeric Separation of Pharmaceutically Important Drug Intermediates
3.1 Introduction
3.2 The Metagenomic Approach
3.3 Lipases as Biocatalysts
3.4 Use of Lipases in Drug Synthesis
3.5 Results
3.5.1 Metagenomic DNA Isolation and Purification
3.5.2 Cloning of Lipase (LipR1) Gene from Soil Sample
3.5.3 Expression and Purification of the LipR1 Protein
3.5.4 Effect of Temperature
3.5.5 Effect of pH
3.5.6 Thermostability Studies
3.5.7 Effect of Different Additives on Lipase Activity
3.5.8 Substrate Specificity
3.5.9 Kinetic Study of the Purified Lipase
3.5.10 Application of This Lipase for Transesterification Reactions
3.6 Use of Ionic Liquids
3.6.1 Reaction with 1-INDANOL
3.6.2 Reaction with (RS)-3-Benzyloxy-1,2-propanediol
3.6.3 Reaction with (RS)-α-Methyl-4 Pyridine Methanol
3.6.4 Reaction with (RS)-α-(Trifluoromethyl) Benzyl Alcohol
3.6.5 Reaction with 1-(1-Naphthyl) Ethanol
3.7 Summary
4. Biotechnological Production of Prenylated Xanthones for Pharmaceutical Use
4.1 Introduction
4.2 Biosynthesis of the Core Structure
4.3 Enzymatic Prenylation of Xanthone Scaffolds in Nature
4.4 Limitations of Chemical Synthesis
4.5 Biotechnological Approaches for in vitro Production of Xanthones
4.5.1 In vitro Cultures
4.5.2 Cascade Biocatalysis: Learning from Nature
4.6 Pharmacological Potential: Effect of Pharmacophores on Cytotoxic Activity of Xanthones
4.6.1 Bioactivities of Chiral Derivatives of Xanthones
4.7 Conclusions
5. Chemoenzymatic Approaches towards (S)-Duloxetine
5.1 Introduction
5.2 Chemoenzymatic Approaches towards (S)-Duloxetine
5.3 Stereoselective Resolution Mediated Synthetic Approaches towards (S)-Duloxetine
5.3.1 Synthesis of (S)-Duloxetine via Immobilized/Mobilized Lipases
5.3.2 Modified Synthesis of (S)-Duloxetine through Dynamic Kinetic Resolution (DKR)
5.4 Stereoselective Reduction Mediated Synthetic Approaches towards (S)-Duloxetine
5.4.1 Synthesis of (S)-Duloxetine via Candida viswanathii
5.4.2 Synthesis of (S)-Duloxetine through Candida pseudotropicalis
5.4.3 Application of Rhodotorula glutinis to Synthesize (S)-Duloxetine
5.4.4 Saccharomyces cerevisiae-Based Synthetic Approach for (S)-Duloxetine
5.4.5 (S)-Duloxetine Synthesis via Candida tropicalis
5.4.6 Synthesis of (S)-Duloxetine by Recombinant Aromatoleum aromaticum
5.4.7 Construction of (S)-Duloxetine Entity via Recombinant Exiguobacterium sp. F42
5.4.8 Synthesis of (S)-Duloxetine through Recombinant Chryseobacterium sp. CA49
5.4.9 (S)-Duloxetine Synthesis via Recombinant Rhodosporidium toruloides
5.4.10 Application of Recombinant Candida albicans to Synthesize (S)-Duloxetine
5.5 Enantioselective Hydrocyanation Mediated Approaches towards (S)-Duloxetine
5.5.1 Synthesis of (S)-Duloxetine via Prunus armeniaca
5.6 Conclusion
6. Synthesis of Antioxidants via Biocatalysis
6.1 Introduction
6.2 What Are Antioxidants?
6.3 Mechanism of Action
6.4 Free-Radical Sources and Implications
6.5 Antioxidants from Biocatalysis
6.5.1 Pure Enzyme Technology
6.5.2 Whole-Cell Biotransformation
6.6 Conclusion
7. Biocatalysts: The Different Classes and Applications for Synthesis of APIs
7.1 Introduction
7.2 Classification of Biocatalysts
7.3 Biocatalysts: Some General Properties
7.4 Enzymes: Mechanisms and Applications
7.4.1 Biocatalysts for Redox Reactions: Mechanisms
7.4.1.1 Applications
7.4.2 Transaminases: Mechanism and Applications
7.4.3 Hydrolases: Mechanism and Applications
7.4.4 Lyases: Aldolases—Mechanism
7.4.4.1 Application in drug design
7.4.5 Hydroxinitrile Lyases
7.5 Conclusion
8. Laccase-Mediated Synthesis of Novel Antibiotics and Amino Acid Derivatives
8.1 Introduction
8.2 Laccases as Mediator for Organic Synthesis
8.3 Enzymatic Transformation of Antibiotics
8.3.1 Phenolic Oxidative Homodimerization
8.3.2 Phenolic Oxidative Heterodimerization
8.3.3 Oxidation Followed by Nuclear Amination
8.3.3.1 para-Dihydroxy aromatic acids and their derivatives aminated by amino-β-lactams
8.3.3.2 ortho-Dihydroxy aromatic acids and their derivatives aminated by amino-β-lactams
8.3.3.3 meta-Dihydroxy aromatics and their reactivity
8.3.3.4 Catechols aminated by amino-β-lactams
8.3.3.5 Alkyl-para-hydroquinones aminated by amino-β-lactams
8.3.3.6 Dihydroxylated aromatics aminated by corollosporines
8.3.3.7 Dihydroxylated aromatics aminated by morpholines
8.3.3.8 Synthesis of mitomycin-like derivatives
8.3.4 Oxidation Followed by Nuclear Thiolation
8.3.4.1 Catechols thiolated by heterocyclic thiols
8.3.4.2 1,4-Naphthohydroquinones thiolated by aryl thiols
8.4 Derivatization of Amino Acids
8.5 Conclusions
9. Hydrolytic Enzymes for the Synthesis of Pharmaceuticals
9.1 Introduction
9.2 Enzymatic Hydrolytic Reactions for the Synthesis of Pharmaceuticals
9.2.1 Hydrolysis of Esters and Amino Esters
9.2.2 Hydrolysis of Amides
9.2.3 Hydrolysis of Epoxides and Nitriles
9.3 Design of Synthetic Transformations over Hydrolysis Processes for the Production of Pharmaceuticals
9.3.1 Esterification of Carboxylic Acids and Acylation of Alcohols and Diols
9.3.2 Acylation Reactions of Amines
9.3.3 Alkoxycarbonylation Reactions
9.4 Conclusions
10. Ene-Reductases in Pharmaceutical Chemistry
10.1 Introduction
10.2 Ene-Reductases: Classification, Substrate Scope, and Reaction Mechanism
10.3 Biocatalytic Applications
10.3.1 Enzyme Engineering
10.3.2 Hydride Sources
10.3.3 Multienzyme Reactions
10.4 Industrial Use of Ene-Reductases
10.4.1 Ene-Reductase Use in the Synthesis of Drugs
10.4.1.1 Profens (2-arylpropanoic acids)
10.4.1.2 Baclofen (β-(4-chlorophenyl)-γ-aminobutyric acid)
10.4.1.3 Pregabalin ((S)-3-(aminomethyl)-5-methylhexanoic acid)
10.4.1.4 Phosphonates
10.4.1.5 Latanoprost
10.4.2 Ene-Reductase Use in the Synthesis of Building Blocks
10.5 Conclusion
11. Biocatalyzed Synthesis of Antidiabetic Drugs
11.1 Introduction
11.2 Insulin and Insulin Analogues
11.3 Amylin Analogues
11.4 Sensitizers
11.4.1 PPAR-α Agonists
11.4.2 Thiazolidinediones (TZDS, Glitazones)
11.4.3 Glitazars
11.5 Insulin Secretagogues
11.6 G Protein-Coupled Receptors Agonists
11.6.1 Incretin Mimetics
11.6.2 GPR119 Agonists
11.7 Enzyme Inhibitors
11.7.1 Dipeptidyl Peptidase-4 (DPP-4) Inhibitors
11.7.1.1 Sitagliptin
11.7.1.2 Saxagliptin
11.7.1.3 Alogliptin, linagliptin, and trelagliptin
11.7.1.4 Teneligliptin and gosogliptin
11.7.1.5 Other DPP4 inhibitors
11.7.2 11β-Hydroxysteroid Dehydrogenase type 1 (11β-HSD1) Inhibitors
11.7.3 α-Glucosidase Inhibitors
11.7.3.1 Iminosugars
11.7.3.2 Carbasugars
11.8 Glycosurics
11.9 Conclusions
12. Glucose-Sensitive Drug Delivery Systems Based on Phenylboronic Acid for Diabetes Treatment
12.1 Introduction
12.2 PBA-Mediated LbL Assembles
12.3 PBA-Regulated Micelles and Vesicles
12.4 PBA-Functionalized Gels
12.5 Conclusion
13. Synthesis of Important Chiral Building Blocks for Pharmaceuticals Using Lactobacillus and Rhodococcus Alcohol Dehydrogenases
13.1 Introduction
13.2 Requirements for Lactobacillus and Rhodococcus ADHs as Versatile Enzymes for the Synthesis of Enantiopure Alcohols
13.3 Alcohols as Chiral Building Blocks Synthesized by Lactobacillus and Rhodococcus ADHs
13.4 Synthesis of Enantiomerically Pure Alcohols with Lactobacillus and Rhodococcus ADHs in Preparative Scale with 1-Phenylethanol as Example
13.5 ADHs from Lactobacillus and Rhodococcus Species in Biocatalytic Cascades
13.6 Searching for New ADHs or Engineering of Well-Known ADHs for Novel Drug Candidates
13.7 Summary
14. Asymmetric Reduction of C=N Bonds by Imine Reductases and Reductive Aminases
14.1 Introduction
14.1.1 Why Are IREDs Important Tools for Biocatalysis
14.1.2 Most Acyclic Imines Have a Low Stability in Aqueous Solutions—Reductive Aminases (RedAm) Solve This Problem
14.1.3 Focus of This Book Chapter
14.2 Imine Reductions Observed in Nature
14.2.1 Imine Reductions of Substrates Bearing a Carboxylate Function
14.2.2 Imine Reductions of Substrates Lacking a Carboxylate Function
14.2.2.1 Alkaloid biosynthesis: IREDs installing an α-chiral amine moiety
14.2.2.2 Alkaloid biosynthesis: IREDs installing a β-chiral amine moiety
14.2.3 Reductive Aminations Observed in Nature
14.3 Imine Reductases Explored for Biocatalytical Imine Reduction
14.3.1 IREDs Belonging to the Hydroxyisobutyrate Dehydrogenases Subfamily
14.3.2 Imine Reduction with Enzymes Belonging to Other Families or Created by Protein Engineering
14.3.3 Scope of Biocatalytic Imine Reduction
14.3.4 Scope of Biocatalytic Reductive Amination: IREDs Require a Large Excess of the Amine Nucleophiles
14.3.5 Reductive Aminases Allow Usage of Near-Stoichiometric Amounts of Amine Nucleophiles for Selected Substrate Combinations
14.3.6 Towards the Synthesis of β-Chiral Amines by Dynamic Kinetic Resolution (DKR) of Aldehydes
14.4 IREDs and RedAms Employed in Cascade Reactions
14.5 Mechanistic Basis of IREDs and RedAms
14.5.1 Structural Features of IREDs Important for Imine Reduction
14.5.2 Mechanistic Differences between Imine Reduction and Reductive Amination
14.6 Conclusive Remarks
15. Cipargamin: Biocatalysis in the Discovery and Development of an Antimalarial Drug
15.1 Introduction
15.1.1 Biocatalysis at Novartis
15.1.2 Malaria and Drug Development
15.2 Biocatalysis in Synthesis of a Drug Candidate
15.3 Biocatalysis in the Synthesis of Metabolites of Cipargamin (KAE609)
15.3.1 Biocatalytic Synthesis of M23
15.4 Biocatalysis in the Synthesis of Cipargamin during Drug Development
15.4.1 Design of a New Route
15.4.2 Biocatalysis Using Kinetic Resolutions
15.4.3 Biocatalysis as a Tool for Asymmetric Synthesis of Chiral Amines
15.4.3.1 Ketone synthesis
15.4.3.2 Transaminase approach
15.4.3.3 Route comparison
15.5 Conclusion
16. Halogenases with Potential Applications for the Synthesis of Halogenated Pharmaceuticals
16.1 Introduction
16.2 Halogenation Mechanisms
16.2.1 Electrophilic Mechanism: Haloperoxidases
16.1.1.1 Heme-iron halogenases
16.1.1.2 Vanadium-dependent alogenases
16.1.1.3 Flavin-dependent halogenases
16.2.2 Radical Mechanism
16.2.3 Nucleophilic Mechanism
16.3 Biosynthesis of Halogenated Pharmaceuticals
16.3.1 Halogenated Pharmaceutical with Antitumor Activity
16.3.2 Halogenated Pharmaceutical with Antibiotic Activity
16.3.3 Halogenated Pharmaceutical with Antifungal Activity
16.4 Perspective
17. Conversion of Natural Products from Renewable Resources in Pharmaceuticals by Cytochromes P450
17.1 Introduction
17.2 Cytochromes P450: General Features
17.2.1 Nomenclature and Classification
17.2.2 Catalytic Cycle of Cytochromes P450
17.3 Cytochromes P450 as Biocatalysts
17.3.1 Importance of Cytochromes P450 in Biocatalysis
17.3.2 Natural Products as a Source of Cytochromes P450 Substrates
17.4 Pharmaceutical Biocatalysis by Cytochromes P450
17.4.1 Synthesis of Statins
17.4.2 Synthesis of Active Steroids
17.4.3 Synthesis of Anticancer Drugs
17.4.4 Synthesis of Antibiotics, Antifungal, and Antiprotozoal Agents
17.4.5 Synthesis of New Natural Product–Inspired Drugs
17.4.5.1 Hydroxy fatty acids
17.4.5.2 Phytopharmaceuticals
17.5 Conclusions and Future Perspectives
18. Oxyfunctionalization of Pharmaceuticals by Fungal Peroxygenases
18.1 Introduction
18.2 Unspecific Peroxygenases
18.2.1 Properties and Occurrence of Unspecific Peroxygenases
18.2.2 Catalyzed Reactions and Reaction Mechanism
18.3 Oxyfunctionalization of Pharmaceuticals
18.3.1 Oxidation of Aliphatics
18.3.2 Oxidation of Aromatics and Olefins
18.3.3 Oxidations Resulting in Cleavage Reactions
18.4 Conclusion and Outlook
19. Biocatalytic Synthesis of Chiral 1,2,3,4-Tetrahydroquinolines
19.1 Introduction
19.2 Enantiomeric Synthesis of 1,2,3,4-Tetrahydroquinoline-4-ols
19.3 Enantiomeric Synthesis of 1,2,3,4-Tetrahydroquinolines
19.4 Conclusion
20. New Strategies to Discover Non-Ribosomal Peptides as a Source of Antibiotics Molecules
20.1 Introduction
20.2 Molecular Mechanism of Antibiotic Resistance
20.3 Nonribosomal Peptides as a Source of New Antibiotics
20.4 Genome Mining Strategies to Find NRPs
20.5 Conclusion
21. Enzyme Kinetics and Drugs as Enzyme Inhibitors
21.1 Introduction
21.2 Enzyme Kinetics
21.2.1 Michaelis–Menten Equation and the Determination of KM and Vmax
21.2.2 Inhibition of Enzymes
21.2.2.1 Competitive inhibition
21.2.2.2 Non-competitive inhibition
21.2.2.3 Uncompetitive inhibition
21.2.2.4 Allosteric modulation
21.2.2.5 Covalent (reversible)inhibition: Pros and cons
21.2.2.6 Ki-/IC50-values and the residence-time model
21.2.3 Enzyme Inhibitors and Activators as Drugs
21.2.3.1 New treatment options for cardiac insufficiency
21.2.3.2 The aldose reductase inhibitor fidarestat enforces chemotherapy
21.2.3.3 Lipid-lowering agents
21.2.3.4 Strategies to combat cancer
21.3 Concluding Remarks
Index
An aparitie | 8 Jan. 2020 |
Autor | Peter Grunwald |
Dimensiuni | 15.49 x 4.06 x 23.11 cm |
Editura | CRC Press |
Format | Hardcover |
ISBN | 9789814800808 |
Limba | Engleza |
Nr pag | 862 |
Clientii ebookshop.ro nu au adaugat inca opinii pentru acest produs. Fii primul care adauga o parere, folosind formularul de mai jos.