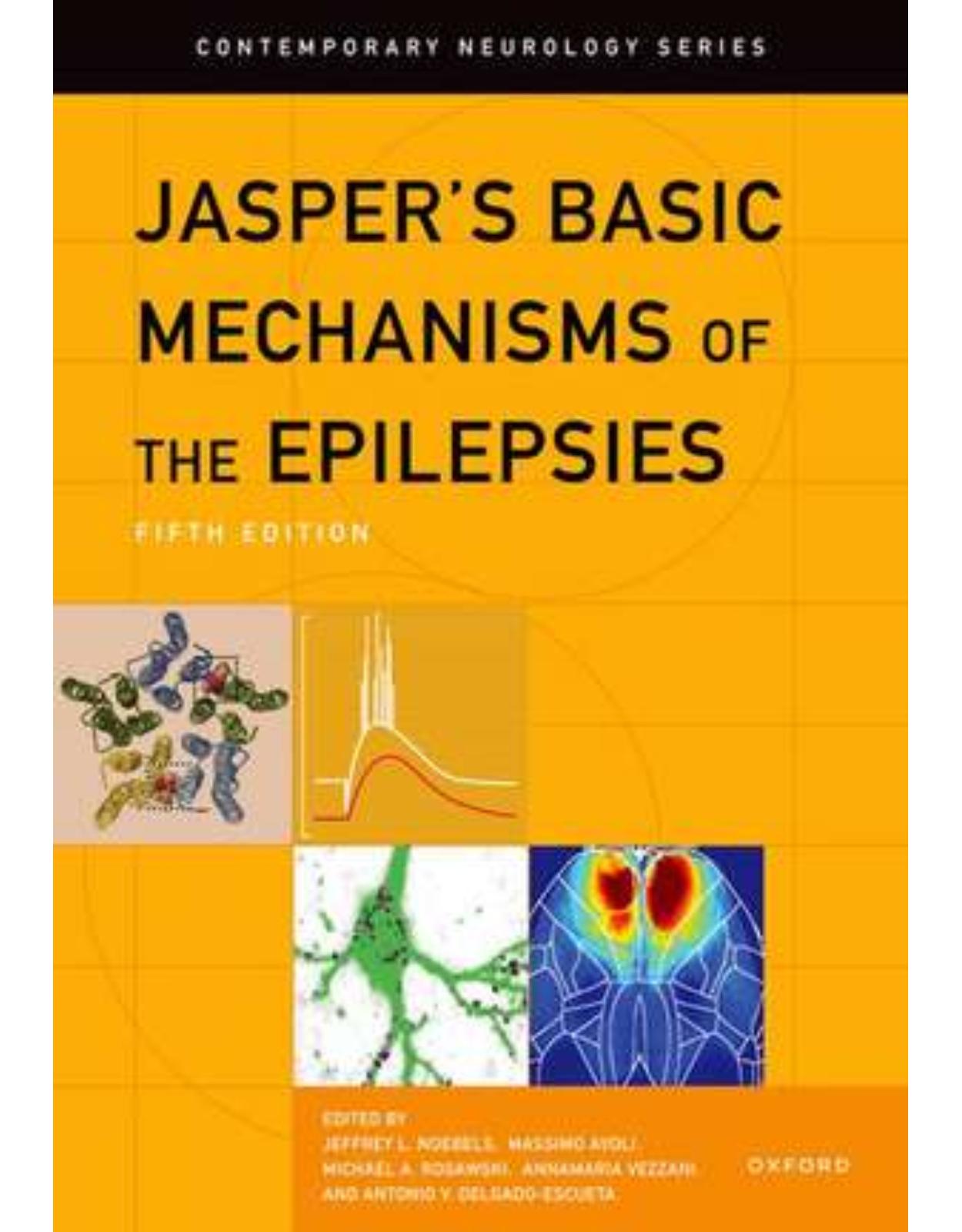
Jaspers Basic Mechanisms of the Epilepsies
Livrare gratis la comenzi peste 500 RON. Pentru celelalte comenzi livrarea este 20 RON.
Disponibilitate: La comanda in aproximativ 4 saptamani
Editura: Oxford University Press
Limba: Engleza
Nr. pagini: 1752
Coperta: Hardcover
Dimensiuni: 188 x 274 x 74 mm
An aparitie: 6 iunie 2024
This is an open access title available under the terms of a CC BY-NC-ND 4.0 International licence. It is free to read at Oxford Academic and offered as a free PDF download from OUP and selected open access locations.Jasper's Basic Mechanisms of the Epilepsies has served as the definitive reference in the field of basic research in the epilepsies for five decades through four well-regarded editions. Since its inception, the book has been an indispensable must-read and belongs in the hands of every experimental epilepsy investigator, practicing epileptologist, clinical neuroscientist, and student for both clinical and basic science reference, doctoral and board exam preparation.This fifth edition is the most ambitious yet and remains the definitive reference in the field, providing encyclopedic and updated coverage of the current understanding of basic research in the epilepsies, while also mapping new research directions for the next decade, and reviewing how molecular laboratory evidence is now being translated into new therapeutics. In 79 chapters, the book considers the role of interactions between neurons, synapses, and glia in the initiation, spread, and arrest of seizures. It examines mechanisms of excitability, synchronization, seizure susceptibility and, ultimately, their contributions to epileptogenesis. It provides a framework for expanding the monogenic epilepsy genome and understanding the complex heredity responsible for common epilepsies. It explores the molecular and cellular disease mechanisms of ion channelopathies, developmental epilepsy genes, and progressive myoclonic epilepsies. It considers newly emerging mechanisms of epilepsy comorbidities. And, for the first time, it describes current efforts to identify biomarkers of disease progression and translate discoveries of epilepsy disease mechanisms into new therapeutic strategies at the frontier of molecular medicine.
Table of Contents:
- Section 1 Evolving Concepts
- 1. The Paroxysmal Depolarizing Shift: The First Cellular Marker of Focal Epileptogenesis
- Introduction
- Neuronal Networks, Synaptic and Intrinsic Membrane Currents, and PDS Generation
- Intrinsic Neuronal Properties and PDS Generation
- Role of Dendritic Ca2+ Spikes in PDS Generation
- Dendritic Abnormalities in Models of Epilepsy
- Concluding Remarks
- 2. Hippocampal Sclerosis in Temporal Lobe Epilepsy: New Views and Challenges
- Introduction
- What Are the Patterns of Cell Loss in Hippocampal Sclerosis?
- What Neurons Remain and How Are They Altered?
- Alterations in Dentate Granule Cells
- Alterations in CA2
- Alterations in the Subiculum
- What Is the Circuitry among Remaining Neurons?
- Future Directions
- 3. Cerebral Cortical Dysplasia or Dysgenesis: Keratan Sulfate Proteoglycan for Fetal Axonal Guidance and Excitatory/Inhibitory Synaptic Targets That Influence Epileptogenesis
- Introduction
- Developmental Principles of Neuroembryology and Cerebral Dysgenesis
- Semantics
- Timing during Ontogenesis
- Developmental Processes
- Neuroblast Maturation
- Keratan Sulfate in Fetal Axonal Guidance
- Keratan Sulfate at Glutamatergic and GABAergic Synaptic Membranes
- Examples of Epileptogenic Dysplasias Influenced by Keratan Sulfate
- Polymicrogyria and Schizencephaly
- Focal Cortical Dysplasia Type Ia
- Examples of Less Epileptogenic or Non-Epileptogenic Disorders Influenced by Keratan Sulfate
- Holoprosencephaly, Alobar, and Semi-Lobar Forms
- Down Syndrome
- Congenital Hydrocephalus
- Atrophic Cerebral Cortex
- Conclusions
- 4. mTOR in Acquired and Genetic Models of Epilepsy
- The mTOR Signaling Pathway
- Clinical Disorders Caused by mTOR Pathway Mutations
- mTORopathy Genes
- Tuberous Sclerosis Complex and Related Malformations of Cortical Development
- Other Syndromes Associated with mTOR
- Clinical Treatment of mTORopathies with mTOR Inhibitors
- Genetic Models of mTORopathies
- An Overview of Models
- General Lessons from Animal Models
- Molecular Mechanisms of Epileptogenesis due to mTOR Hyperactivation
- Ion Channel/Neurotransmitter Regulation
- Inflammatory Mechanisms
- Metabolism and Autophagy
- Morphological and Pathological Effects of mTOR Hyperactivation on Cell Structure
- Genetic Effects: Not All mTORopathy Genes Are Equal
- mTOR Hyperactivation in Acquired Epilepsy
- Challenges and Opportunities
- 5. Epilepsy Genomics: Disease-Causing Sequence Variants
- Introduction
- History of Curating the Epilepsy Genome: The First Ten Years of Epilepsy Variants
- Benefits of Curating the Epilepsy Genome
- Genetic Testing for Epilepsy Variants Improves Diagnosis Treatment and Prognosis
- Novel Molecular Concepts of Epileptogenesis in Genetic Epilepsy Syndromes
- Epilepsy Genomics: At Last A Road to Halting and Reversing Disease Progression and Possible Cures
- Key Elements in the Genetic and Clinical Platform Needed in the Quest to Cure Each Epilepsy
- Key Turning Points
- Remaining Challenges and Trends in the Next Ten Years
- Ex Vivo HSPC Transduction Gene Therapy Can Cure MLD
- Genome Editing in Hurler Mucopolysaccharidosis I
- Lafora Type Progressive Myoclonic Epilepsy: The Key Elements and Critical Genetic and Clinical Ingredients Necessary for a Quest to Cure Are Already Available
- Developmental and Epileptic Encephalopathies
- Focal Epilepsies
- Vigilance for Unexpected Adverse Effects of ASOs and GRT
- Key Social and Ethical Issues in the Quest to Cure the Epilepsies
- Section 2 Seizures, Networks, and Systems
- 6. GABA-Receptor Signaling and Ionic Plasticity in the Generation and Spread of Seizures
- Introduction
- Network Patterns of Focal Seizures
- Interneurons and Epileptiform Events: A Prelude
- Focal Seizure Patterns: From Epilepsy Patients to Animal Models In Vivo and In Vitro
- Activity-Dependent Ionic Mechanisms Underlying Fast Transformation from Inhibitory to Excitatory GABAR Signaling
- Ionic Basis of the Reversal Potential and Driving Force of GABAR-Mediated Currents
- High-Frequency Activity of Interneurons Leads to GABAR-Mediated Excitation
- Recurrent Epileptiform Events Driven by GABARs in the Absence of Glutamatergic Transmission
- Ionic Avalanches in the Generation and Spread of Seizures
- Glutamate-Induced Depolarization Enhances Neuronal GABAR-Mediated Cl− Loading
- Positive Feedback Loops Involving Ion Shifts and Epileptiform Activity
- Cellular and Molecular Sources of K+ Contributing to Activity-Dependent K+ Transients
- Chronic Epilepsy and Long-Term Ionic Plasticity
- Conclusions
- 7. Connexins, Pannexins, and Epilepsy
- Introduction
- Fundamental Biology of Gap Junctions, Connexins, and Pannexins
- Gap Junctions and Connexins
- Pannexin Channels
- Connexin-36 and Pannexin-1 in Neurons
- Connexins and Pannexins in Glia
- Connexins and Pannexins in the Neuro-Glial-Vascular Unit
- Gap Junctions and Epilepsy
- Gap Junctional Function and Seizures
- Alterations of Gap Junction Coupling in Brain Specimens of Epileptic Patients and Relevant Animal Models
- Glial-Based Gap-Junctional-Mediated Extracellular Potassium Homeostasis and Seizures
- Pannexins and Seizures
- Conclusions
- 8. Mechanisms Leading to Initiation, Development, and Termination of Focal Seizures
- Introduction
- Focal Seizure Patterns in Humans
- Focal Seizure Patterns in Animal Models
- Chronic Animal Models of Focal Epilepsy In Vivo
- In Vitro Models of Focal Seizures
- Focal Seizure Onset
- Progression of a Focal Seizure Discharge
- Focal Seizure Termination
- The Relationship between Focal Seizure Termination and Postictal Suppression
- Conclusions
- 9. Transition to Seizure from Cellular, Network, and Dynamical Perspectives
- Introduction
- Seizure Initiation
- Cellular and Network Mechanisms of Seizure Initiation
- Transition to the Seizure and Preictal State
- The Phenomenon of Critical Slowing and Loss of Resilience
- Experimental and Empirical Evidence for a Critical Slowing and Loss of Stability in the Epileptic Brain
- Empirical Evidence for a Preictal Critical Slowing and Loss of Stability in Humans
- Proictal States, Seizure Probability Fluctuation, and Markers of Resilience
- Conclusions and Future Research
- 10. Role of the Subiculum in Focal Epilepsy
- Introduction
- Subiculum: Anatomy, Local Networks, and Cellular Properties
- Anatomy within the Brain and Links with Temporal Lobe Cortices
- Subiculum Substructures
- Local Neuronal Networks
- Neuron Subtypes: Morphology and Electrophysiological Properties
- Subiculum Physiology: More Than a Hippocampal Output
- Subiculum Local Oscillatory Rhythms
- Memory
- Spatial Orientation
- Stress Response
- Role of the Subiculum in Rodent Models of Epilepsy
- In Vivo Involvement of the Subiculum in Focal Epilepsy
- In Vitro Indication of the Role of the Subiculum in Focal Epilepsy
- Role of the Subiculum in Human Focal Epilepsy
- Ex Vivo Experimental Data from Human Epileptic Postoperative Tissues
- In Vivo Evidence of the Subicular Role in Temporal Lobe Epilepsy
- Conclusions
- 11. Optogenetic Modulation of Focal Seizures
- Introduction
- Optogenetic Stimulation in In Vitro Models of Epileptiform Synchronization
- Optogenetic Stimulation in In Vivo Models of Mesial Temporal Lobe Epilepsy
- Acute Seizures
- Spontaneous Seizures
- Limitations and Clinical Translation of Optogenetic Procedures
- Concluding Remarks
- 12. Balancing Seizure Control with Cognitive Side Effects Using Changes in Theta
- Introduction
- Network Approach to Epileptic Seizures
- Pathological Theta and Relationship to Interneurons Preictally
- Pathological Theta-Related Decrease in Neuronal Activity Interictally
- Pathological Theta and Reduced Cognitive Performance
- Stimulation of Theta Reduces Ictogenesis and Attenuates Cognitive Dysfunction
- Impact of an Inclusive Framework on Treatment
- 13. High-Frequency Oscillations
- Definitions
- Recording Methods
- Separating Physiological from Pathological HFOs
- Basic Mechanisms of Generation
- Are There Different Mechanisms in Neocortex?
- Visual Analysis and Automatic Detection in Clinical Settings
- Relationships to the Epileptogenic Zone
- Fast Ripples and the Underlying Anatomopathological Entities
- Biomarker of Epilepsy
- Conclusion
- 14. Seizures and Sleep
- Neuronal Activity during Sleep Oscillations and Epileptic Activity
- Bidirectional Interactions between Epileptic Activity and Sleep
- Relationship between Epileptic Activity and Sleep
- Distribution of Seizures across the Sleep-Wake Cycle
- High-Frequency Oscillations are Modulated by Sleep
- Sleep-Related Hypermotor Epilepsy as an Example of a Sleep-Related Epilepsy Syndrome
- Sleep Microstructure and Epileptic Activity
- Link between Sleep Fragmentation and Epileptic Activity
- Localizing Value of Sleep for Seizure Focus Identification and Outcome Prediction
- Effects of Sleep Homeostasis and Circadian, Multidien, and Circannual Rhythms of Epileptic Activity
- Effects of Sleep-Related Epileptic Activity on Sleep Structure and Function
- SUDEP, Seizures, and Sleep
- Future Directions
- 15. Cycles in Epilepsy
- Introduction
- Historical Perspective
- Ancient Beliefs
- Epilepsy Colonies
- The Invention of the EEG
- Chronic Recordings in Animals
- Methodological Advances
- Digital Seizure Diaries
- Ambulatory Recording Devices
- Computational Advances
- Sleep-Wake and Circadian Seizure Cycles
- Sleep-Wake Seizure Cycles
- Circadian Seizure Cycles
- Circadian Seizure Networks
- Circadian Seizure Timing in Animal Models
- Combined Circadian and Sleep-Wake Modulations
- Putative Mechanisms
- Multidien Seizure Cycles
- Multidien Cycles of Seizures
- Multidien Cycles of Epileptic Brain Activity
- The Relationship between Seizures and IEA
- Free-Running Rhythms
- Putative Mechanisms
- Circannual Seizure Cycles
- Putative Mechanisms
- Impact and Future Challenges
- 16. Human Single-Neuron Recordings in Epilepsy
- Introduction
- Devices for Recording Single Neurons in Humans
- Behnke-Fried Hybrid Depth Electrodes
- “Utah” Microelectrode Arrays
- Recording Methodology and Technical Considerations
- Ethical Considerations
- Recording Techniques
- Single- and Multi-Unit Discrimination and Analyses
- Multi-Unit Activity and Spike-Sorting Single Units
- Cell-Type Subclassification
- Insights into Ictal Dynamics from Human Single-Neuron Recordings
- Spatiotemporal Activity of Human Neurons during Seizures
- Tracking Single-Unit Activity during Seizures: Special Considerations
- Correlating Single-Neuron Data with Clinical Recordings
- Cell-Type-Specific Activity during the Ictal Transition
- Translating Animal Studies to Human Single-Unit Recordings
- Human Single-Neuron Activity in the Interictal Period
- Single-Neuron Neurocognitive Studies in Epilepsy Patients
- Future Considerations
- 17. Role of Ion Concentration Dynamics in Epileptic Seizures
- Introduction
- Potassium Ions and the K+ Accumulation Hypothesis
- Sodium Ions and the Na+/K+ Pump
- Chloride
- Calcium and Magnesium
- Conclusion
- 18. A Classification of Seizures Based on Dynamics
- Introduction
- A Taxonomy of Seizure Dynamics
- Prelude
- Seizures and Bifurcations
- The Dynamotypes
- Application to Clinical Data
- Summary of Observed Dynamotypes
- Noise and Complex Dynamics
- Coexistence of Dynamotypes
- Implications for the Clinic
- Conclusion
- 19. Computational EEG Analysis of Human Epileptogenic Networks
- Introduction
- Scalp EEG
- Intracranial Stereo-EEG
- Epileptogenicity Index
- Statistical Parametric Mapping of Epileptogenicity Index
- Quantified Frequency Analysis Index
- Nonlinear Structure Index
- High-Frequency Oscillations
- Ultra-Long-Term EEG
- The Next Ten Years
- 20. Excitation-Inhibition Balance in Absence Seizure Ictogenesis
- Intrinsic Mechanisms
- T-Type Ca2+ Channels
- HCN Channels
- Summary
- Network/Synaptic Mechanisms
- Summary
- SWD Frequency in Animal Models and Humans
- Conclusions
- 21. Cortical and Thalamic PV+ Interneuron Dysfunction in the Pathogenesis of Absence Epilepsy
- The Corticothalamic Circuit Involved with Absence Epilepsy
- Brief History of Thalamocortical Inhibition in Absence Epilepsy
- Evolution of Available Models of Absence Epilepsy
- Debate on the Thalamic versus Cortical Onset of Seizures
- Genetic and Molecular Insights into Cell-Type-Specific Contributions to Absence Epilepsy
- Insights from Drug-Induced Models of Absence Seizures
- Insights from Monogenic Models
- Further Insight from Inbred Rat Models of Absence Epilepsy
- A Working Model of Absence Epileptogenesis
- Insights into Network Mechanisms from Recent In Vivo Imaging and Minimally Invasive Manipulation Studies
- In Vivo Imaging Studies
- Optogenetic/DREADD Studies
- New Directions and Treatment Considerations
- Conclusions
- 22. Convergence of Thalamic Mechanisms in Genetic Epilepsies
- Introduction
- Thalamic Organization and Rhythmogenesis
- Structural Elements of Thalamic and Thalamocortical Circuits: From Gross Anatomy to Cell Types
- Thalamic Firing
- Calcium Channels and Thalamic Firing
- Calcium Channels
- Spotlight on T-Type Calcium Channels and Burst Firing
- Spatial Distribution of T-Type Calcium Channel Subunits
- Rhythmogenesis in the Thalamus: Strengths and Weaknesses
- Delta Oscillations
- Spindle Oscillations
- Slow Oscillations
- The Thalamus in Absence Epilepsy and Beyond
- Insights from Human Genetic Studies of Absence Epilepsy
- T-Type Calcium Channels in Genetic Models of Absence Epilepsy
- R-Type and P/Q-Type Channels in Genetic Models of Absence Epilepsy
- SK Channels and Thalamic Bursting in Dravet Syndrome
- The Emerging Role of the Thalamus in Acquired Epilepsies
- Conclusions
- Section 3 Epileptogenesis: Molecular Mechanism and Treatments
- 23. The Diverse Roles of Mossy Cells in the Normal Brain, Epileptogenesis, and Chronic Epilepsy
- Introduction: Defining Mossy Cells
- Thorny Excrescences as a Defining Feature—With a Caveat
- Somatic and Dendritic Characteristics
- Axon
- Neurotransmitter
- Cell-Specific Markers of MCs
- Electrophysiology
- Dorsal and Ventral MCs
- Summary
- Functional Role of MCs in the Normal DG
- Inputs and Outputs of MCs in the Normal DG
- Are MCs Excitatory or Inhibitory to GCs?
- Plasticity
- Hippocampal EEG
- Behavior
- MCs in Epileptogenesis and Epilepsy
- How Studies of MCs in Epilepsy Led to Predictions about Their Role in the Disease
- How Studies of the Normal Brain Suggested Additional Ways MCs Influence Epileptogenesis and Epilepsy
- Advent of New Methods Using Mice Expressing Cre in MCs and AAV
- The Era of Mouse Models of Epilepsy: What It Has Suggested about the Role of MCs
- Caveats and Open Questions
- 24. Temporal Lobe Epileptogenesis: A Focus on Etiology, Neuron Loss, the Latent Period, and Dentate Granule Cell Disinhibition
- Introduction
- Three Steps to Refractory Temporal Lobe Epilepsy When Prolonged Febrile Seizures Are an Antecedent Factor
- Excitotoxicity, Neuron Loss, Disinhibition, and Temporal Lobe Epileptogenesis
- The Etiology of Hippocampal Sclerosis
- Prolonged Excitation Can Damage the Normal Brain; No Preexisting Defect Is Needed
- The Conundrum of Granule Cell Disinhibition without Dentate Basket Cell Degeneration
- The “Dormant Basket Cell” Hypothesis
- Extending the “Dormant Basket Cell” Hypothesis; Lateral Inhibition in the Normal and Injured Dentate Gyrus
- The Latent Period; When after Injury Do Self-Generated Epileptic Seizures Begin?
- The Definition of “Epileptogenesis” and the Notion of the “Gestational” Latent Period
- Is Neuron Loss Immediately Epileptogenic or Does Epilepsy Require Time, Reactive Gliosis, and Tissue Shrinkage (Sclerosis)?
- Is the Latent Period after Nonconvulsive Status Epilepticus a State of Clinically Subtle Seizures or of No Seizures at All?
- Is Dentate Gyrus Disinhibition a Directly Epileptogenic Mechanism?
- GABAergic Disinhibition Instantly Triggers Acute “Nonepileptic” Seizures, but Could Disinhibition Also Cause Spontaneous “Epileptic” Seizures?
- Can GABA Neuron Ablation Cause Prolonged Seizures, Hippocampal Sclerosis, and Epilepsy?
- A Unifying Theory of Inherited and Acquired TLE Epileptogenesis
- Step One: Initiation of Epileptogenesis
- Step Two: Injury, When It Occurs
- Step Three: Epileptogenesis; What Is It and When Does It Occur?
- Unaddressed and Unanswered Questions
- Synopsis
- 25. Adult Neurogenesis in Epileptogenesis and Comorbidities
- Concept of Epileptogenesis
- Morphological Changes of Adult-Born Granule Cells in Epilepsy
- Molecular Regulators of Aberrant Neurogenesis in Epilepsy
- Glial Control of Aberrant Neurogenesis in Epilepsy
- Functional Roles of Adult Hippocampal Neurogenesis in Acute and Chronic Phase of Epilepsy
- The Role of Normal Newborn Granule Neurons in Generation of Acute Seizures
- The Role of Newborn Granule Neurons in Epileptogenesis
- Role of Aberrant Neurogenesis in Epilepsy-Associated Comorbidities
- Conclusions and Future Perspectives
- 26. A Crucial Role for Astrocytes in Epileptogenesis: Gap Junctions and Glutamate Receptors
- Introduction
- Gap Junction Channels
- The Astroglial Network and Its Potential Role in Epilepsy
- Connexin Expression and Gap Junctional Coupling in Human and Experimental Epilepsy
- Impact of Genetic and Pharmacological Modulation of Gap Junctions on Neuronal Excitability and Epileptogenesis
- Glutamate Receptors
- Glutamate Uptake Dysregulation in Epileptogenesis
- Regulation of Metabotropic Glutamate Receptors in Epileptogenesis
- Targeting Astrocyte Glutamate Receptors as a Therapy for Refractory Epilepsies
- Conclusions
- 27. Adenosine Kinase: Cytoplasmic and Nuclear Isoforms
- Introduction
- The Biochemistry of Adenosine: Role in Epilepsy
- ADK-S Regulates Extracellular/Synaptic Adenosine
- ADK-S Regulates Cytoplasmic Adenosine Levels and Transmethylation Reactions
- Nuclear ADK-L Regulates Epigenetic Mechanisms
- Adenosine Kinase Hypothesis of Epileptogenesis
- Targeting ADK for Epilepsy Treatment
- Targeting ADK-S and Elevating Extracellular Adenosine for Seizure Suppression
- Targeting ADK-L for Epilepsy Prevention
- Conclusions and Therapeutic Perspective
- 28. Inflammatory Astrocytic TGFβ Signaling Induced by Blood–Brain Barrier Dysfunction Drives Epileptogenesis
- Introduction
- The Blood–Brain Barrier
- Physiological BBB Function
- Dysfunctional BBB
- BBBD in Epileptogenesis
- BBBD Induces Inflammatory TGFβ Signaling
- Astrocytic TGFβ Activation Induces Functional Network Modifications, ECM Remodeling, and Pathological Synaptic Plasticity
- Translational Impact—Therapeutic Approaches to BBB Dysfunction in Epilepsy
- Diagnostics: Detecting BBBD as a Biomarker in Epileptogenesis
- BBBD and TGFβR as Targets for Antiepileptogenic Interventions
- Conclusions
- 29. Pericytes and Microglia: Neurovascular and Immune Regulatory Cells in Seizure Disorders
- Introduction: Neuro-Glio-Vascular Regulatory Cells in Seizure Networks
- What Is a Pericyte?
- Perivascular Inflammatory Cell Reactivity during Seizures: Focus on Pericytes
- What Are Microglial Cells?
- Microglia Surveillance and Neuronal Interactions
- Microglial Pro- and Anti-inflammatory Molecular Equilibriums in Experimental Epilepsy
- Microglia-Pericytes Perivascular Assembly and Reactivity during Seizures: Experimental and Clinical Evidence
- Microglial Profiles in Human Drug-Resistant Epilepsies
- Pharmacological Entry Points: Focus on Pericytes and Microglia
- Conclusion: Refining Timing and Targets for Pharmacological Interventions
- 30. Neuroinflammation in Epilepsy: Cellular and Molecular Mechanisms
- Introduction
- Evidence for Neuroinflammation as a Risk Factor and Intensifying Influence for Epilepsy
- Neuroinflammatory Pathways Relevant to Epilepsy
- Cytokines
- Cytokine Mechanisms Underlying Hyperexcitability and Neurotoxicity
- Interleukin-1β
- Direct Neuronal Effects Relevant for Seizures
- Indirect Effects on Neuronal Excitability Mediated by Glia and Brain Endothelium
- Tumor Necrosis Factor
- Interaction with Glutamatergic and GABAergic Neurotransmission
- Other Neuromodulatory Effects
- Effects Mediated by Glial Cells
- IL-6
- Inflammation-Induced Channelopathies: Impact on Neuronal Excitability and Comorbidities
- Lipopolysaccharide
- Polyinosinic:Polycytidylic Acid
- COX-2 Signaling Pathways
- EP1 Receptors Mediate P-glycoprotein Induction in the Blood–Brain Barrier
- EP2 Receptors Exacerbate Neuroinflammation
- Chemokines
- CXCL1
- CCL2-CCR2
- Complement Pathway
- Mechanisms Underlying Complement Effects
- Variants in Immune-Related Genes
- Conclusions
- 31. Role of Reactive Oxygen Species in Epilepsy
- Introduction
- Neuron-Glial Interactions and Their Role in Metabolic Dysfunction Associated with Seizures
- Role of Oxidative and Nitrosative Stress in Epilepsy
- Redox Homeostasis
- Sources of Reactive Oxygen Species Production
- Redox-Mediated Cellular Pathway Disruption in Epilepsy
- Redox Regulation of Nrf2 in Epilepsy
- Biomarkers
- Therapeutic Strategies
- Conclusions
- 32. BDNF/TrkB Signaling and Epileptogenesis
- Introduction
- BDNF and TrkB Biology
- BDNF/TrkB Signaling: Epileptogenesis Caused by Trauma
- BDNF/TrkB Signaling: Development of Epilepsy Caused by Hypoxic/Ischemic Insults
- BDNF/TrkB Signaling: Development of Epilepsy Caused by Seizures
- BDNF/TrkB Signaling: A Role in Neuronal Survival
- Summary and Perspective
- 33. Clinical Features and Molecular Mechanisms Underlying Autoantibody-Mediated Seizures
- Introduction
- Clinical Features of Antibody-Mediated CNS Disorders
- NMDA Receptor Antibody Encephalitis
- LGI1-Antibody Encephalitis
- Other Autoantibody-Mediated Syndromes
- An Enduring Tendency to Seizure?
- Immunopathogenesis
- Neuropathogenesis
- Mechanisms of Autoantibodies Directed against Synaptic Receptors
- Mechanisms of Antibodies Directed against Other Extracellular Proteins
- Conclusions
- 34. Transcriptomic Alterations in Epileptogenesis: Transcription Factors in the Spotlight
- Introduction
- Transcriptional Control by FOS and JUN
- Transcriptional Control by Early Growth Response Genes
- Transcriptional Control by Serum Response Factor
- Transcriptional Control by the CREB Signaling Pathway
- Transcriptional Control Mechanisms by SP1
- Inflammation-Associated Transcriptional Regulation by NF-kB
- The JAK/STAT Signaling Cascade
- Zn2+-Induced Transcriptional Control
- Circadian Clock-Controlled Transcription
- NRF2-Mediated Control of Antioxidant Defenses
- Transcriptional Repression by RE1-Silencing Transcription Factor
- Genetic Variants and Transcriptional Control Mechanisms
- Differential Transcriptional Regulation by Alternative Promoters
- Summary and Future Course
- 35. Epigenetics
- Introduction
- Basic Concepts
- Genomes Are Nonrandomly Spatially Organized in 3D
- Readers, Writers, and Erasers
- Cellular Memory
- Key Epigenetic Mechanisms
- DNA Methylation
- Histone Modifications
- Noncoding RNAs
- Chromatin Remodeling
- On the Epigenetic Origin of Epilepsy
- Epigenetics in Epileptic Encephalopathies
- DNA Methylation in Focal Epilepsy
- Noncoding RNAs in Focal Epilepsy
- Histone Methylation in Focal Epilepsy
- Histone Acetylation in Focal Epilepsy
- A Key Role for Metabolism
- Epigenetic Biomarkers in Epilepsy
- Outlook
- Section 4 Biomarkers of Epileptogenesis
- 36. EEG Biomarkers of Epileptogenesis
- The Electroencephalogram
- EEG as a Biomarker for Epileptogenesis
- EEG Signatures of Epileptogenesis
- Interictal Spikes
- High-Frequency Oscillations
- Theta-Wave Dynamics
- Nonlinear Dynamics
- Future Challenges and Potential Mitigation Strategies
- Epilepsy Is Not One Disorder
- Translating Animal Findings into Clinical Tools
- Future Opportunities
- More Efficient Clinical Trials
- More Effective Treatments
- Wearable EEG
- Conclusions
- 37. Blood Biomarkers: Noncoding RNAs and Proteins
- Introduction
- The Challenge of Diagnosis and Prognosis
- What Is a Biomarker and Why Are Circulating Blood Molecules Sought?
- Practical Uses of a Circulating Biomarker
- Why Should Circulating Biofluids Contain Molecular Biomarkers of Epilepsy?
- Other Criteria That Must Be Met for Circulating Molecular Biomarkers
- What Type of Molecules Should We Be Looking for?
- How Would a Molecular Biomarker Be Used?
- miRNAs as Epilepsy Biomarkers
- miRNAs—An Overview
- miRNAs as Biomarkers
- Presence of miRNAs in Blood in Epilepsy—Clinical Findings
- Presence of miRNAs in Blood in Epilepsy—Preclinical Model Findings
- Practical Issues—How Will miRNA Biomarkers Be Detected?
- Other Circulating Noncoding RNAs as Biomarkers of Epilepsy
- Circulating Protein Biomarkers of Epilepsy
- Circulating Structural Protein Biomarkers of Epilepsy
- Circulating Inflammation-Related Protein Biomarkers of Epilepsy
- Current Gaps—What We Know We Don’t Know
- Summary and Conclusions
- 38. Behavioral Biomarkers of Epileptogenesis and Epilepsy Severity
- Introduction
- Neurobehavioral Comorbidities of Epilepsy: Bidirectional Relationship
- Shared Neuropathological Mechanisms between Epilepsy and Comorbidities
- Behavioral Comorbidities as Biomarkers of Epileptogenesis
- Behavioral Comorbidities as Biomarkers of Epilepsy Severity
- Conclusions and Future Directions
- 39. Genetic and Imaging Biomarkers of Epileptogenesis
- Introduction
- Biomarker Types
- Genetic Biomarkers
- Genetic Risk Biomarkers
- Diagnostic Genetic Biomarkers
- Prognostic Genetic Biomarkers
- Predictive Genetic Biomarkers
- Imaging Biomarkers
- Imaging Risk Biomarkers
- Human Studies
- Diagnostic Imaging Biomarkers
- Prognostic Imaging Biomarkers
- Predictive Imaging Biomarkers
- Conclusions
- 40. Machine-Learning Approach to Discover Novel Biomarkers for Posttraumatic Epilepsy
- Introduction
- Materials and Methods
- Animals and Experimental Procedures
- Data Preprocessing and Feature Engineering
- Exploratory Data Analysis and Statistical Hypothesis Testing
- Machine Learning
- Model Training and Evaluation
- Cohort Similarity Assessment
- Implementation
- Results
- Exploratory Data Analysis and Hypothesis Tests
- Classification
- Subcohort Similarity
- Discussion
- Section 5 Genes and Network Development
- 41. Human Epilepsy Gene Discovery: The Next Decade
- Genetic Contributions to Epilepsy: Current Knowledge
- Genetic Generalized Epilepsy
- Focal Epilepsy
- Developmental and Epileptic Encephalopathy
- What’s Next?
- Genome Sequencing
- Long-Read Sequencing
- Epigenetics
- Multiomics
- Mosaicism
- Oligogenic and Polygenic Risk
- The Importance of Collaboration
- Summary
- 42. Functional Exploration of Epilepsy Genes in Patient-Derived Cells
- Introduction
- Generating iPSCs
- Gene Editing of iPSCs
- Methods of 2D Neuronal Differentiation
- Physiological Assays for hPSC-Derived Neurons
- Genetic Epilepsy Modeling Using 2D hPSC Cultures
- Dravet Syndrome
- STXBP1-Related DEE
- Tuberous Sclerosis Complex
- Rett Syndrome
- Studying SUDEP Using hPSCs
- Brain Organoid Models
- Modeling Genetic Epilepsies and Their Effects on Cortical Network Function With Brain Organoids
- Challenges and Future Directions
- Conclusions
- 43. Brain Mosaicism in Epileptogenic Cortical Malformations
- Introduction
- Somatic Mutations in mTOR Pathway Genes in Focal Cortical Dysplasia
- Brain Somatic Mutations in the N-Glycosylation Pathway in MOGHE
- Modeling of Brain Somatic Mutations in Rodents
- In Utero Electroporation to Model Somatic Mosaicism
- Genetic Hyperactivation of mTOR to Model FCDII
- Cellular and Circuit Features Underlying Focal Cortical Dysplasia
- Human Studies
- Mouse Studies
- Disease Modeling Using Human Stem Cell Models
- Precision Medicine and Perspectives
- 44. Sodium Channelopathies in Human and Animal Models of Epilepsy and Neurodevelopmental Disorders
- Introduction
- SCN1A
- SCN1A Variants in Patients with Epilepsy and Neurodevelopmental Disorders
- In Vitro Analyses of Nav1.1 Mutant Channels
- Nav1.1 Distribution in Brain
- SCN1A Animal/Human Cell Models and Disease Pathogenesis
- Precision Therapies for Diseases with Nav1.1 Deficiency
- SCN2A
- SCN2A Variants in Patients with Epilepsy and Neurodevelopmental Disorders
- In Vitro Analyses on Nav1.2 Mutant Channels
- Nav1.2 Distribution in Brain
- SCN2A Animal Models and Disease Pathogenesis
- SCN8A
- SCN8A Variants in Patients with Epilepsy and Neurodevelopmental Disorders
- In Vitro Analyses of Nav1.6 Mutant Channels
- Nav1.6 Distribution in Brain
- Scn8a Animal Models and Disease Pathogenesis
- SCN1B
- SCN1B Variants in Patients with Epilepsy and Neurodevelopmental Disorders
- In Vitro Analyses of β1 and β1B Wild-Type and Mutant Channels
- SCN1B Expression in Brain
- SCN1B Animal Models and Disease Cascade
- Mechanisms of SUDEP in SCN1B Animal Models
- Concluding Remarks
- 45. Potassium Channels in Genetic Epilepsy: A Functional Perspective
- Introduction
- Brief Summary of Potassium Channel Classification
- Characterization and Classification of Potassium Channel Variants
- Types of Potassium Currents in Neurons
- IA-Related Channelopathies and Epilepsy (KCND2, KCND3)
- ID-Related Channelopathies and Epilepsy (KCNA1, KCNA2)
- IK-Related Channelopathies and Epilepsy (KCNB1, KCNC1)
- IM and ImAHP-Related Channelopathies and Epilepsy (KCNQ2, KCNQ3, KCNQ5)
- IfAHP-Related Channelopathies and Epilepsy (KCNMA1, KCNB2)
- Slow Afterhyperpolarization (sAHP)-Related Channelopathies and Epilepsy (KCNT1, KCNT2, KCNQ2, KCNQ3, KCNJ11)
- Ikir-Related Channelopathies and Epilepsy (KCNJ10, KCNJ11)
- Ileak-Related Channelopathies and Epilepsy (KCNK4)
- KCNH1-Related Channelopathies and Epilepsy
- New Concepts, Gaps in Our Knowledge, and Novel Approaches
- Potassium Channels, Developmental Expression, and Homeostasis
- Potassium Channels as Signaling Hubs
- Potassium Channels—Cotransporter Complexes
- Large-Scale Approaches to Bridge the Gap between Potassium Channel Dysfunction and Seizures
- Conclusion
- 46. High-Voltage-Activated Calcium Channels in Epilepsy: Lessons from Humans and Rodents
- Introduction
- Voltage-Gated Calcium Channels: An Overview
- L-Type VGCCs in Epilepsy
- L-Type Ca1.2 Channels: CACNA1C-Associated Disorders in Humans
- L-Type Ca1.3 Channels: CACNA1D-Associated Disorders in Humans
- Mechanisms of L-Type VGCC-Associated Epilepsy: Dendritic Calcium Transients, Afterhyperpolarization, and Paroxysmal Depolarization Shifts
- P/Q-Type Ca2.1 VGCC and Epilepsy
- P/Q-Type Ca2.1 Channels: CACNA1A-Associated Disorders in Humans
- Physiological Roles of P/Q-Type Ca2.1 Channels
- Cellular and Circuit Mechanisms of Cacna1a-Associated Epilepsy
- R-Type Ca2.3 Channels: CACNA1E-Associated Epilepsy
- CACNA1E-Associated Disorders in Humans
- Mechanisms of CACNA1E-Related Epilepsies: Ca2.3-Mediated R-Type Currents Regulate Firing Mode, Plateau Potentials, and Afterhyperpolarization
- Conclusions
- 47. Transcriptional Regulation of Cortical Interneuron Development
- Introduction
- Subpallial Progenitor Domain Subdivisions
- Medial Ganglionic Eminence
- Caudal Ganglionic Eminence
- Lateral Ganglionic Eminence
- Pre-Optic Area
- Septum
- Regional Specification of IN-Generating Progenitor Zones
- MGE Specification and LGE/CGE Identity Repression
- Roles of Gsx1 and Gsx2 in Establishing LGE and CGE
- Ventral and Rostral Patterning Centers Together Induce Nkx2.1 and the MGE through SHH and FGF8 Signaling
- IN Fate Mapping
- Identification of MGE-Derived Descendants
- Identification of CGE-Derived Descendants
- POA-Derived INs
- Pallial Progenitors Contribute to Olfactory Bulb IN Diversity
- Shh Fate Mapping
- Fgf8 and Fgf17 Fate Mapping
- Progenitor Zones/Stem Cell Biology in the Ganglionic Eminences
- GE Ventricular Zone
- GE Subventricular Zones
- Mechanisms Proposed for the Generation of SST and PV CIN
- Functions of TFs Expressed in Migratory and Post-Migratory Immature INs
- Postmitotic Roles of Dlx TFs in IN Survival, Morphogenesis, and Synapse Formation and Function
- Nr2f1/Nr2f2 Promotes CGE Fate Specification and MGE Generation of SST CIN
- Role of Arx in MGE-Derived IN Migration and PV IN Fate Specification
- Opposing Roles of Npas1 and Npas3 in the MGE/CGE-Derived IN Generation and Differentiation
- Roles of the Mafb and c-Maf TFs in PV/SST IN Fate Specification, Migration, and Maturation
- Activity-Dependent Expression of Satb1 Drives SST CIN Maturation
- Mef2c Controls PV CIN Differentiation
- Genomic Approaches to Understand Transcriptional Control of IN Development
- Forebrain Enhancer Identification and Functional Characterization
- Epigenetic Functions of Dlx1/2/5, Gsx2, Lhx6, Nkx2.1, and Otx2 during GE Development
- Molecular and Genetic Tools Utilizing Enhancer
- Interneuron Classification and New Marker Discovery through Single-Cell RNA Sequencing
- Conclusion
- 48. GABA Receptors, Seizures, and Epilepsy
- GABA Receptors in Epilepsy and as Therapeutic Targets
- GABAR Structure
- GABAR Subtypes
- Targeting GABAR Subtypes for Epilepsy Therapy
- Tolerance
- Treatment of Epilepsy Comorbidities
- EtOH-Induced Plasticity of GABAR-Mediated Inhibition at the Gene Expression and Protein Levels
- Synaptic Matrix Tethering with Neuroligin-2, Gephyrin, and Collybistin; Role of LHFPL4
- Activation of Extrasynaptic δ-GABAR Induces Spike-Wave Seizures
- Advances in GABAR Structural Pharmacology Relevant to Pentobarbital, Propofol, and Etomidate
- Neuroactive Steroids
- Benzodiazepines
- Stiripentol
- Optogenetics and Chemogenetics
- Conclusion and Future Directions
- 49. Gene–Genome Interactions: Understanding Complex Molecular Traits in Epilepsy
- Complex Molecular Networks in Epilepsy
- The Growing Epilepsy Genome
- Studying the “Multiome”
- Single-Cell Analysis
- Single-Cell Genomics
- Single-Cell Transcriptomics
- 3D Genome Conformation
- Genetics 3.0—Artificial Intelligence and Deep Learning
- Better Phenotypes, Faster
- A Normative Framework for Complex Data––From Computer Vision to Genomic Sequence
- New Phenotypes from Neural Networks
- The Next Ten Years
- Section 6 Progressive Myoclonus Epilepsies
- 50. The Neuronal Ceroid Lipofuscinoses
- Introduction
- Genetics
- Epidemiology
- Clinical Features
- Neuroimaging and EEG Findings
- Pathology
- Morphology
- Molecular Basis of Disease
- NCL Proteins
- Diagnosis
- Laboratory Diagnosis
- Prenatal Diagnosis
- Care Management
- Therapy
- Enzyme Replacement Therapy for CLN2 Disease
- Experimental Therapies
- Conclusions and Future Directions
- 51. Progressive Myoclonus Epilepsy: Unverricht-Lundborg Disease
- Introduction
- Clinical Features
- Genotype-Phenotype Correlations
- Differential Diagnosis
- The Cystatin B Gene and Protein
- Disease-Associated CSTB Mutations
- Cystatin B–Deficient Mouse Model for EPM1
- Disease Mechanisms
- Regulation of Histone Cleavage, Cell Cycle, and Neurogenesis
- GABAergic Signaling and Synapse Physiology
- Microglial Dysfunction and Inflammation
- Oxidative Stress and Apoptotic Cell Death
- 52. Strategies on Gene Therapy in Progressive Myoclonus Epilepsies
- Introduction
- Molecular/Genetic Markers
- Gene Therapy with Particularities to Progressive Myoclonus Epilepsy
- Unverricht-Lundborg Disease
- Lafora Disease
- Neuronal Ceroid Lipofuscinoses
- Gene Replacement Therapy for NCLs
- Conclusion
- 53. Therapeutic Window for the Treatment of Lafora Disease
- Brain Glycogen
- Lafora Disease
- Pathological Contribution of Glycogen in the LD Brain
- Targeting Glycogen to Treat LD
- Malin Gene Replacement Therapy to Treat LD
- New Challenges and Perspectives Using MGS-Based Suppression or Malin-Restoration Approaches
- Conclusions
- 54. Progressive Myoclonus Epilepsy of Lafora: Treatment with Metformin
- Introduction
- Proposed Mechanism of Action of Metformin
- Inhibition of Mitochondrial Glycerol-3-Phosphate Dehydrogenase
- Inhibition of the Lysosomal Proton Pump v-ATPase
- Inhibition of Mitochondrial Complex I of the Respiratory Chain and Activation of AMP-Activated Protein Kinase
- Metformin Ameliorates Oxidative Stress
- Metformin and Neuroinflammation
- Metformin as a Neuroprotective Agent in Epilepsy
- Metformin in Lafora Disease
- Clinical Aspects of Lafora Disease
- Animal Models of Lafora Disease
- Pharmacological Interventions in Animal Models of Lafora Disease
- 55. Treating Lafora Disease with an Antibody-Enzyme Fusion
- The Problem: Lafora Bodies Drive Epilepsy, Neurodegeneration, and Inflammation
- Glycogen
- Synthesis and Degradation
- Glycogen: Architecture
- Glycogen and LBs in the Brain
- The Solution: Novel Antibody-Enzyme Fusions Clear LBs
- Enzymatic Degradation of LBs
- The 3E10 Targeting Platform
- VAL-0417 Activity in Cells and Systemic Administration
- CNS Administration of VAL-0417
- VAL-0417 Ablates Brain LBs
- Assessing Brain Function via Metabolomics
- Metabolic Profiles to Assess Target Engagement
- Brain Glycogen: Glucose and Glucosamine
- Next Steps
- 56. Antisense Oligonucleotide Therapy for Progressive Myoclonus Epilepsies
- Progressive Myoclonus Epilepsies—A Brief Overview
- Antisense Oligonucleotides and Their Different Modes of Action
- ASO Strategies to Treat PME Disorders
- ASOs That Modulate Splicing
- ASOs Targeting mRNA for Degradation via RNase H
- Upregulation of Gene Expression through ASOs
- Combination of ASOs with Readthrough Drugs to Target Nonsense Mutations
- Conclusions and Future Perspectives
- Section 7 Comorbidities of Epileptic Networks
- 57. Dissecting Epileptic and Cognitive Network Dysfunction in Epilepsy
- Introduction
- Memory Processes
- Measuring the Functional Integrity of Neuronal Networks
- The Output: Behavioral Assays
- Probing Brain Functions through Single-Unit Activity
- Brain Rhythms: Networks in Motion
- Functional Mechanisms of Cognitive Impairment in Epilepsy
- Epilepsy Models
- Neuronal Dynamics and Coding in Epilepsy
- Development of Neural Coding and Oscillations
- GABAergic Neurons: Coordinators of Complex Systems
- Dissecting Epileptic and Cognitive Network Dysfunction
- Conclusion and Perspectives
- 58. Attention-Deficit Disorders and Epilepsy
- Epilepsy and ADHD—Clinical Background and Genetics
- Epidemiology of Comorbid ADHD and Epilepsy
- Genetics of ADHD and Epilepsy
- Pathophysiologic Insights from Rodent Models of Epilepsy and ADHD
- Testing Attention Deficits in Rodents
- Rodent Models of ADHD and Epilepsy/Seizures
- Insights into Current and Future Treatment of Attention Disorders in Epilepsy
- The Effect of Pharmacotherapy for ADHD on Seizures
- Treatment of Concurrent Seizures and Attention Disorders
- Future Screening of Antiseizure Drugs
- Conclusion
- 59. What Rodent Models Teach Us about the Association of Autism and Epilepsy
- The Association of Autism and Epilepsy
- The Role of Animal Models for Studying Autism/Epilepsy Syndromes
- Dravet Syndrome: An Ion Channelopathy Causing Refractory Seizures, Cognitive Deficits, and Autism
- Calcium Channelopathies
- Potassium Channels: Kv4.2 and Kv7.2
- CNTNAP2
- Do Seizures during Development Cause Impairments in Social Behavior?
- Future Directions
- 60. Artificial Intelligence–Guided Behavioral Phenotyping in Epilepsy
- Introduction
- Why Do We Care about Behavior in Epilepsy?
- What Kind of Behavioral Readout and Expertise Is Required to Phenotype Animal Models of Epilepsies?
- Analyzing Behavior Starts with Tracking
- Machine Learning and Deep Learning Revolutionized Animal Motion Tracking
- With Machine Learning Toward Marker-less Animal Tracking
- Basics of Deep Learning
- State-of-the-Art Animal Motion Capture with Deep Learning
- Quantifying Behavior
- Reference Coordinates
- Decomposing the Temporal Structure of Behavior
- Conclusion and Future Directions for Basic Epilepsy Research
- AI-Guided Phenotyping in Epilepsy for Screening at Scale
- Linking Brain to Behavior in Epilepsy with AI-Guided Phenotyping
- 61. Mechanisms of Depression in the Epileptic Brain
- Epilepsy and Depression Comorbidity
- Phenomenology
- Management and Treatment
- Depression and Vulnerability to Epilepsy
- Epilepsy and Vulnerability to Depression
- Mechanisms of Depression
- Potential Mechanisms Mediating Comorbid Depression and Epilepsy
- Network/Structural Abnormalities
- HPA Axis
- Altered Neurotransmission
- Summary
- 62. Heterogeneous Mechanisms of Spreading Depolarization and Seizures
- Introduction
- SD Initiation/Propagation
- SD Contributors and Pharmacosensitivity
- Roles of Astrocytes
- Neuronal Structural Alteration and Injuries in SD
- Physiological and Pathological SD Repolarization
- Neurovascular Responses in SD and Seizure
- Anatomical Susceptibility of SD
- SD and Seizure Generation in Acute, Subacute, and Chronic Conditions
- Hypoxia/Ischemia/Hypoglycemia
- Brain Trauma/Mechanical Stress
- Hyperthermia/Fever
- SD Generation/Inhibition by Seizure Activities
- Clinical Associations between Seizure and SD
- SD Recording in Human Epilepsy Patients
- Summary
- 63. Genetic and Cellular Mechanisms Underlying SUDEP Risk
- Introduction
- The MORTEMUS Study Defines a Common Temporal Framework for Nocturnal Sudden Death
- The MORTEMUS Pattern and Timetable Have Mechanistic Implications
- Ictal Asystoles, Ictal Apneas, and Postictal Cortical EEG Suppression Are Unreliable SUDEP Biomarkers
- Ictal Asystoles
- Ictal Apneas
- Postictal Generalized EEG Suppression
- Atypical SUDEP Patterns: Parallels with SCD, SIDS, and SUDY
- Monogenic SUDEP Risk
- The Neurocardiac Gene Hypothesis for SUDEP
- Potassium Channels
- KCNQ1
- KCNA1
- SENP2
- Other
- Sodium Channels
- SCN1A
- SCN1B, 2A, 8A
- Ryanodine Receptor
- RYR2
- SUDEP Gene Diversity
- Monogenic Neurorespiratory Syndromes
- Gene-Specific Longevity Profiles: SD50
- Epistatic Interactions among SUDEP Genes Impact Survival
- Conditional Genetic Dissection of Critical SUDEP Pathways
- Heart versus Brain
- Forebrain versus Brainstem
- Excitatory versus Inhibitory Networks
- Progressive Central and Cardiac Pathology and SUDEP Risk
- Forebrain
- Brainstem
- Cardiac
- Diurnal Rhythm and SUDEP
- Brainstem Spreading Depolarization
- Brainstem SD Is Linked to Postictal Cardiorespiratory Collapse in Mouse SUDEP Models
- In Vivo SD Imaging
- Seizure-SD Coupling
- The Perilous Genetic Landscape of SUDEP
- SUDEP: Gene-Guided Research and Interventions
- Circuitry
- Modifier Genes
- Pharmacology
- Gene Therapy
- Summary
- Section 8 Epilepsy Therapeutics
- 64. New Models for Assessment of Antiseizure Activity
- Introduction
- Zebrafish and Other Model Organisms
- Drosophila melanogaster Models of Epilepsy
- Mouse Models of Genetic Epilepsy and Therapy Development
- Theiler’s Murine Encephalomyelitis Virus Mouse Model
- Intra-Amygdala Kainate and Intra-Hippocampal Kainate Mouse Models
- Induced Pluripotent Stem Cells and the Future
- Summary and Conclusions
- 65. Disease Biology Factors Accounting for Epilepsy Severity: An Updated Conceptual Framework for New Drug Discovery
- Call for Paradigm Shift Toward Treating Disease Biology
- Drug-Resistant Epilepsy: Time to Break with Traditional Views
- Concept of Intrinsic Epilepsy Severity Index
- Contribution of the Epileptic Perturbation to the Intrinsic Epilepsy Severity Index
- Contribution of Mitigating and Propagating Factors to Intrinsic Epilepsy Severity
- Contribution of Recurrent Seizures to Intrinsic Epilepsy Severity
- Future Technologies Will Drive Better Understanding of Neurobiological Factors Contributing to the Epilepsy Severity Index
- Intrinsic Disease Severity Concept Provides a Holistic View to Drug Resistance
- Targeting Intrinsic Epilepsy Severity as an Approach to Achieve Disease Control
- Conclusion
- 66. Animal Models of Pharmacoresistant Epilepsy
- Introduction
- 6 Hz Psychomotor Seizure Model
- Lamotrigine-Resistant Kindled Rodent Model
- Phenytoin-Resistant Kindled Rat Model
- Post–Status Epilepticus Models of Spontaneous Recurrent Seizures
- Intra-Amygdala and Intra-Hippocampal Kainate SE
- Systemic Kainate- and Pilocarpine-Induced-SE Model
- Electrical Stimulation Induced-SE Model
- Conclusion
- 67. Drug Combinations for Antiepileptogenesis
- Introduction
- Single Drug versus Drug Combinations for Antiepileptogenesis
- Efficacy of Drug Combination to Prevent or Modify the Development of Epilepsy
- Systematic Evaluation of Drug Combinations for Antiepileptogenesis
- Three Drug Combinations Stand Out in Their Antiepileptogenic Efficacy
- Potential Mechanisms of Effective Drug Combinations
- Effects on Diverse versus Similar Targets for Antiepileptogenesis
- Top-Down versus Bottom-Up Target-Based Approaches in Identifying New Antiepileptogenic Therapies
- Future Advancements in the Search for Synergistic Antiepileptogenic Drug Combinations
- Conclusions and Outlook
- 68. Prophylaxis of Epileptogenesis in Injury and Genetic Epilepsy Models
- Gabapentinoids, Excitatory Synapse Formation, and Antiepileptogenesis
- Antiepileptogenesis in a Genetic, Noninjury Model of Epilepsy with Enhanced Excitatory Connectivity
- Antiepileptogenesis in Posttraumatic Epilepsy and a Genetic Epilepsy Model with Reduced Inhibitory Interneuronal Function
- Unresolved Issues
- 69. Management of Febrile Status Epilepticus: Past, Present, and Future
- Introduction
- Outcomes Following Febrile Status Epilepticus: Risk of Epilepsy
- Outcomes Following Febrile Status Epilepticus: Cognitive Deficits
- Pharmacologic Management of FSE: Past and Present
- Neuroimaging after FSE to Predict Clinical Outcomes
- Future Treatments to Prevent Long-Term Neurological Changes Following FSE
- The Future of FSE and Its Treatment
- 70. Excitatory Transmission in Status Epilepticus
- Introduction
- Cholinergic Agents and Glutamate Analogs Induce Status Epilepticus
- Glutamate Receptors
- Glutamate Receptor Expression in the Brain
- Glutamate Receptor Plasticity during SE
- Glutamate Receptor Antagonists in the Treatment of SE: Studies in Experimental Animals
- Glutamate Excitotoxicity and Cell Death
- NMDA Receptors Regulate the Plasticity of GABA-A and AMPA Receptors during SE
- NMDA Receptors Regulate Epileptogenesis
- Glutamate Receptor Antagonists in the Treatment of SE
- Conclusions
- 71. Ionic Mechanisms of Ictogenic Disinhibition: All GABA Signaling Is Local
- Introduction
- Physiology
- The GABA Reversal Potential
- E versus RMP
- When Are GABA Currents Excitatory?
- Is E a Monolithic Number?
- What Determines E?
- The Role of Transporters in Chloride Homeostasis
- All GABA Signaling Is Local
- Cytoplasmic Chloride Microdomains
- Role of Transporters in the Chloride Distribution by Displacement Model
- Experimental Analysis of Chloride Distribution by Displacement
- Pathology
- Seizures Associated with Acute Brain Injury
- Salt Flux Associated with Neuronal Injury
- Other Mechanisms of Salt Influx
- Bumetanide Trials for Neonatal Seizures
- Human Bumetanide Trials
- Temporal Variance in E
- Spatial Variance in E
- Chronic Epilepsy
- 72. Epileptogenic Channelopathies Guide Design of NBI-921352, a Highly Isoform-Selective Inhibitor of Na1.6
- Introduction
- Na Cellular and Subcellular Distribution
- Central Nervous System
- Peripheral Tissues
- Genetic Channelopathies Guide a Preferred Selectivity Profile for Antiseizure Medications
- Peripheral Nas
- Na1.1
- Na1.6
- Designing a New Class of Isoform-Selective Sodium Channel Inhibitors
- NBI-921352, the First Isoform-Selective Inhibitor of Na1.6
- Like Nonselective Na Inhibitor ASMs That Bind the Pore Domain, NBI-921352 Is a State-Dependent Inhibitor
- NBI-921352 Inhibits Persistent and Resurgent Currents from Mutant Na1.6 Channels
- NBI-921352 Inhibits Electrically Induced Seizures in Scn8aN1768D/+ Mice
- Concentration Dependence of NBI-921352 in Comparison to Common ASMs
- NBI-921352 Inhibits Electrically Induced Seizures in Wild-Type Mice and Rats
- Efficacious Concentrations of NBI-921352 Are Well Separated from Concentrations That Provoke Behavioral Signs
- In Vitro Na1.6 Inhibition Predicts In Vivo Efficacy
- Conclusions
- 73. Purinergic Signaling in Epilepsy
- Purinergic Signaling in the Brain
- ATP Receptors
- Adenosine Receptors
- Interconversion of Endogenous Purinergic Ligands
- Sources and Release
- Targeting Purinergic Receptors for Seizure Control and the Treatment of Epilepsy
- ATP Release during Seizures and Epilepsy
- Targeting Adenosine and P1 Receptors
- P2 Receptors
- P2 Receptor–Mediated Molecular Mechanisms Contributing to Seizures and Epilepsy
- Purines as Diagnostics for Epilepsy
- Conclusion
- 74. Anti-inflammatory Strategies for Disease Modification: Focus on Therapies Close to Clinical Translation
- Introduction
- The Need for Biomarkers
- Anti-inflammatory Treatments in Clinical Practice
- Interference with the IL-1beta-IL-1R1 Axis
- IL-1beta-IL-1R1 Axis Activation in Epilepsy
- Clinical Studies with Anti-IL-1beta Treatments
- Arachidonic Acid and COX-2 Signaling Pathways
- Timing of Intervention
- Disease Modification as Clinical Target
- Dexamethasone
- Interference with Leukocyte-Endothelial Cell Interaction by Natalizumab
- Statins
- Combinatorial Anti-inflammatory Therapy
- Summary and Conclusions
- 75. Targeted Augmentation of Nuclear Gene Output (TANGO)
- Dravet Syndrome: An Intractable Developmental and Epileptic Encephalopathy
- Identification of Nonsense-Mediated Decay, or Poison, Exons, in SCN1A
- TANGO: A Therapeutic Strategy That Takes Advantage of NMD Exons
- Noncoding Sequences in SCN1A
- Other Therapeutic Strategies on the Horizon
- SCN8A ASO
- Viral Approaches
- Rett Syndrome and Angelman Syndrome—Other DEEs with Unique Challenges
- Rett Syndrome
- Angelman Syndrome
- Pros and Cons of Gene Regulation Therapy
- Timing of Treatment
- Concerns with Viral Vectors
- Concerns with ASO Treatment
- GOF Variants
- Administration of Treatment
- Study Planning
- Health Economics
- Conclusion
- 76. Gene Therapy for Epilepsy
- Introduction
- Genetic Epilepsy
- Clinical Trials in Genetic Epilepsy
- Gene Therapy
- Overview of Gene Therapy
- Types of Gene Therapy
- Viral Vectors
- Current Limitations of Gene Therapy
- Gene Therapy in Epilepsy
- Gene Replacement
- Gene Editing and Manipulation
- Delivery of Neuroactive Substances
- Conclusion
- Key Points
- 77. Gene Therapy for Refractory Epilepsy
- Introduction
- Viral Vectors
- Promoters
- Transgenes: Manipulation of the Excitation/Inhibition (E/I) Balance
- Regulated Gene Therapy
- Upregulation of Endogenous Gene Expression
- Neurotrophic Factors
- Monogenic Epilepsies
- Clinical Translation
- Conclusions
- 78. Cell Therapy for Treatment of Epilepsy
- Introduction
- The Route to Clinical Studies
- Proof-of-Concept Studies
- Delivering GABA: Cell Resources and Progress
- Initial Studies to Engineer Cells for GABA Release
- Animal Sources of Inhibitory Neurons
- Human Stem Cells
- Clinical Considerations
- Functional Properties of IN
- Preclinical Studies with Cell Transplantation
- Antiseizure Efficacy in Experimental Models of Epilepsy
- Functional Integration into the Host Brain
- Histology
- Other Disease-Modifying Effects
- Safety
- Where Are We Now?
- Conclusion
- 79. Mechanisms of Ketogenic Diet Action
- Introduction
- Historical and Clinical Perspectives
- Early Animal Models
- Mechanistic Studies in the Early Renaissance Era
- Ketone Bodies and Glucose
- Neurotransmitters and Neuromodulators
- Fatty Acids
- Mitochondria, Anaplerosis, Oxidative Stress, and Redox Imbalance
- Insights at the Close of the First Century of Use
- Neuroinflammation
- Gut Microbiome
- Epigenetic Regulation
- Beyond Epilepsy
- 2021 National Institutes of Health Ketogenic Diet Workshop
- Conclusions
- Index
An aparitie | 6 iunie 2024 |
Autor | Jeffrey L. Noebels, Massimo Avoli, Michael A. Rogawski, Annamaria Vezzani, Antonio V. Delgado-Escueta |
Dimensiuni | 188 x 274 x 74 mm |
Editura | Oxford University Press |
Format | Hardcover |
ISBN | 9780197549469 |
Limba | Engleza |
Nr pag | 1752 |
-
1,57300 lei 1,30000 lei
Clientii ebookshop.ro nu au adaugat inca opinii pentru acest produs. Fii primul care adauga o parere, folosind formularul de mai jos.